Monitoring temperature variability inside a healthcare facility during an extreme heat event using low-cost sensors
Introduction
Individual extreme heat events (EHEs) can be associated with hundreds to thousands of excess deaths (1) and higher rates of emergency department visits (2). Since the 1950s, climate change has led to more frequent and severe EHEs globally, and they are expected to become more common in upcoming decades. To protect public health, it is important to develop a strong understanding of the risks associated with EHEs, particularly in facilities occupied by people who are susceptible to experiencing adverse health outcomes from exposure to high temperatures.
Certain individual-level characteristics are associated with increased susceptibility to the health effects of EHEs. For example, older age, disabilities, and other pre-existing conditions put individuals at elevated risk of heat-related illness and death during EHEs (3). Healthcare facilities house patients who may be particularly susceptible to the impacts of extreme heat, so it is important to understand how temperatures within these facilities are affected by EHEs. Healthcare workers may have additional risk factors that make them more vulnerable to heat exposure, such as performing physically demanding tasks while wearing personal protective equipment that may reduce their ability to shed heat. Indoor temperatures experienced throughout a healthcare facility may vary depending on the use of mechanical cooling (i.e., air conditioning), the floor, and the size and orientation of the windows (4-6). Variability in temperatures may be particularly pronounced in older facilities that were constructed under obsolete (or non-existent) building codes and may be more likely to have outdated cooling systems (7,8). However, there is limited published information on how indoor temperatures are affected by and change during EHEs at healthcare facilities where people may be particularly susceptible to heat.
The aim of this study was to explore the range of temperatures experienced by patients and staff inside an older healthcare facility in Vancouver, British Columbia (BC) during an unprecedented EHE in the summer of 2021. We examine temperature time series data from low-cost air quality sensors co-located inside and outside of this facility as well as data from a nearby weather monitoring station. Our objectives were to describe temperature variation during the EHE: (I) across multiple locations inside the healthcare facility; (II) between sensors inside and outside of the facility; and (III) between the sensors and a nearby weather monitoring station.
Methods
Study context
Vancouver has a temperate climate, with historical [1971–2000] maximum (max.) daily temperatures in June approximately 14–24 ℃ (9). In late June 2021, BC experienced an unprecedented period of extreme hot weather which resulted in temperature anomalies 16–20 ℃ above season norms. Analyses early after this event concluded that this was an EHE that would have been virtually impossible without human-induced climate change (10). In this study, the EHE period was defined as 24 June to 02 July, 2021 because this was the period in which temperatures were elevated above season norms (11) and corresponds with previous reports on the health impacts of this EHE (12,13).
The study site was a large inpatient rehabilitation center in Vancouver. The building was constructed in 1972 and has four floors and a basement. Services on the ground floor include physio- and occupational therapy, cafeterias, and offices while floors 2–4 contain all patient wards. The basement houses maintenance rooms, storage, and manufacturing facilities. The building has central air conditioning and operable windows. Satellite imaging (Google, California) from June 2021 shows a moderate amount of green space and trees around the building which may provide some shading to the northwest and southeast corners. However, no sensors were located in those areas of the facility (Figure 1).
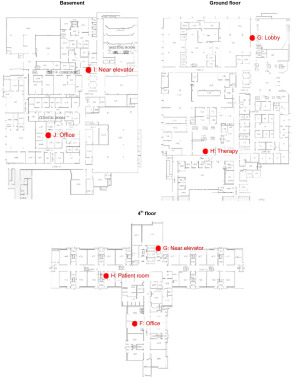
Air temperature sensors
Nine Air Quality Egg sensors (EGGs) (Wicked Device, New York) were installed at the facility in August 2020 as part of another study on air quality (14). Two EGGs were installed outdoors—one on the fourth-floor roof and one outside a second-floor window—and seven were installed indoors in the basement (N=2), on the ground floor (N=2), and on the fourth floor (N=3). In the basement, one was installed near the elevator and one in a side hallway. On the ground floor, one was installed in a central therapy room and one in the lobby. On the fourth floor, one was installed near the elevator, one in a patient room, and one in an office. EGGs recorded temperatures in one-minute intervals during the EHE. The temperature sensors (Sensirion SHT35) are accurate to 0.2 ℃ and have an operating range of −40 to 125 ℃.
Nearby air monitoring station
We compared EGG measurements to data from a nearby weather station located less than four kilometers away from the study site, operated by the Metro Vancouver Regional District (15). This station was chosen because it (I) was the nearest weather station to our study site; (II) was a similar distance to the coast; and (III) was used in the previous study on air quality at this facility (14). Hourly temperature data was available from this weather station.
Descriptive statistics
Average temperatures were calculated: (I) individually for each EGG; (II) across all EGGs on the same floor; and (III) across all indoor and outdoor EGGs separately. Averages were computed for each day and for the entire EHE. Means were compared among sensors using either a two-sample t-test or analysis of variance (ANOVA) with follow-up Tukey post-hoc test, as appropriate for comparison of two or more means.
Results
Outdoor versus indoor temperatures
Outdoor temperatures during the EHE at the facility were higher than those recorded indoors and at the nearby weather station (Figure 2; Table 1). Outdoor temperatures recorded at the facility reached a max. of 47.6 ℃ around 16:00 at the EGG outside the second-floor window. The mean temperature during the EHE across both outdoor EGGs was 27.2 ℃ and the minimum (min.) was 15.9 ℃, which was recorded on the fourth-floor roof. Indoor temperatures across all EGGs reached their peak approximately three hours after the outdoor peak (min.: 20.1 ℃, mean: 23.6 ℃, max.: 32.2 ℃). Temperatures at the nearby weather station reached their max. at the same time as the outdoor EGGs but were up to 10.4 ℃ lower than the outdoor EGGs (min.: 16.1 ℃; mean: 25.3 ℃; max.: 37.2 ℃). The mean temperature across all indoor EGGs (23.6 ℃) was significantly (P<0.001) lower than both the mean temperature across outdoor EGGs (27.2 ℃) and the mean temperature at the nearby weather station (25.3 ℃).
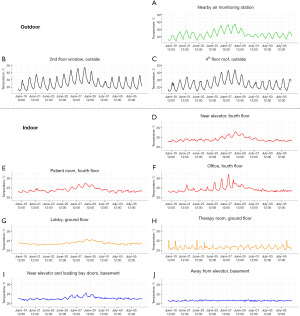
Table 1
Location | Mean (range, SD) (℃) | Date maximum temperature (time of day) |
---|---|---|
Outdoors | ||
Nearby weather station | 25.3 (16.1–37.2, 5.17) | 2021-06-28 (19:00) |
2nd floor window | 28.0 (17.0–47.6, 7.27) | 2021-06-28 (15:54) |
4th floor roof | 26.5 (15.9–44.4, 6.71) | 2021-06-28 (16:50) |
4th floor | ||
Office | 25.2 (23.0–32.2, 1.67) | 2021-06-27 (18:42) |
By elevator | 24.8 (22.9–28.2, 1.25) | 2021-06-28 (20:11) |
Patient room | 24.7 (22.6–27.6, 1.15) | 2021-06-28 (18:28) |
Ground floor | ||
Lobby | 24.2 (22.8–26.0, 0.73) | 2021-06-28 (23:09) |
Therapy | 21.5 (20.1–25.4, 0.65) | 2021-06-24 (06:22) |
Basement | ||
By elevator | 23.3 (22.0–25.9, 0.79) | 2021-06-28 (20:07) |
Away from elevator | 21.7 (21.1–22.3, 0.20) | 2021-06-30 (15:17) |
EHE, extreme heat event; SD, standard deviation.
Variability between floors
Indoor temperatures varied between floors (Figure 2). Temperatures among the three EGGs on the fourth floor were most affected by the EHE, with the sensor in the fourth-floor office reaching a max. temperature of 32.2 ℃ on 27 June. The mean across all sensors on the fourth floor was 24.9 ℃ and the min. was 22.6 ℃ in the patient room. The EGGs on the ground floor (min.: 20.1 ℃, mean: 22.8 ℃, max.: 26.0 ℃) and the basement (min.: 21.1 ℃, mean: 22.5 ℃, max.: 25.9 ℃) never recorded temperatures higher than 26 ℃. Average daily temperatures across all EGGs on same floor were all significantly different between floors (P<0.001).
Variability between rooms on the same floor
Rooms on the same floor were also affected differently by the EHE (Figure 2), such that average daily temperatures were all significantly different among EGGs on the same floor (P<0.001). In the basement, the EGG located by the elevator and the loading bay door increased in temperature during the hottest periods of the day (min.: 22.0 ℃, mean: 23.3 ℃, max.: 25.9 ℃), while the EGG located away from the elevator was unaffected (min.: 21.1 ℃, mean: 21.7 ℃, max.: 22.3 ℃). On the ground floor, the lobby was the most impacted by the EHE with temperature increasing from 24 June until declining with outdoor temperature after 29 June (min.: 22.8 ℃, mean: 24.2 ℃, max.: 26.0 ℃). In comparison, the ground-floor therapy room has its own climate control system and had lower max. temperatures during the EHE (min.: 20.1 ℃, mean: 21.5 ℃, max.: 25.4 ℃) than it did in the week before (min.: 19.9 ℃, mean: 21.5 ℃, max.: 25.6 ℃) and after the event (min.: 20.1 ℃, mean: 21.5 ℃, max.: 25.7 ℃).
The top floor of the building is shaped like a cross, with one arm extending in each cardinal direction (Figure 1). Temperatures on this floor increased steadily during the EHE, with the EGG in a west-facing office in the south arm having the most extreme highs and lows (min.: 23.0 ℃, mean: 25.2 ℃, max.: 32.2 ℃). The EGG near the fourth-floor elevator (min.: 22.9 ℃, mean: 24.8 ℃, max.: 28.2 ℃) was located away from major exterior walls (Figure 1). The third EGG on this floor was located inside of a patient room and had the lowest temperatures (min.: 22.6 ℃, mean: 24.7 ℃, max.: 27.6 ℃).
Discussion
In this study we described air temperature outside and inside a large older inpatient healthcare facility in BC during an unprecedented EHE in the summer of 2021. We found that indoor temperatures remained substantially lower than outdoor temperatures, but that temperature varied considerably between floors and between locations on the same floor, despite central air conditioning.
Overall, this study highlights how indoor temperatures may vary across a single healthcare facility during an EHE. There are many potential explanations for why we observed different temperatures throughout this facility. For example, the fourth (top) floor was markedly hotter than the basement and ground floor. This is consistent with previous research which has found increasing temperatures with higher floors (16,17) and may result from cumulative heat transfer upwards from lower floors and solar gain through the roof (18). There was also variation between rooms on the same floor. For instance, the fourth-floor office with a west-facing window reached temperatures 4.5 ℃ hotter than the max. temperature in the fourth-floor patient room with a south-facing window. Factors affecting different indoor temperatures are complex and may be the result of interactions between occupant behavior, mechanical cooling, window size and cardinal orientation, room size, building material, and building age (4-6). Lower daily max. temperatures at the nearby weather station compared with the outdoor sensors at the facility may reflect outdoor sensor placement (e.g., in full sun, on a black rooftop surface), or differences in the urban heat island effect (19,20). These results suggest that areas within the same building may experience different temperatures during EHEs due to differing designs, surroundings, and behaviours of users (4,17,20). The differences in involved factors can be difficult to model across different buildings because they change over time and space (21,22), but their impacts on temperature can easily be measured and monitored in real-time.
Although most studies have focused on the impacts of hot outdoor temperatures, there is a growing body of evidence demonstrating indoor temperature thresholds and exposure times at which adverse health impacts, such as heat strain, heat stress, sleep disturbance, fatigue, and headache may start becoming more likely among susceptible populations (23). Recent work (24,25) monitoring core body temperatures, blood pressure, and heart rate in older adults in controlled laboratory settings have reported that sustained exposure (≥8 hours) to temperatures between 26 and 31 ℃ may pose a risk in some adults, while sustained exposure to temperatures over 31 ℃ should be avoided by susceptible populations. The ASHRAE guidelines for temperature in non-surgical rooms, which consider not only patient safety, but also infection control, and occupant satisfaction, are even lower, at 21–24 ℃ (26,27). In this study, temperatures on the fourth floor where patients were housed exceeded all these temperature thresholds, potentially exposing susceptible people to dangerous temperatures.
The exact health risks associated with specific indoor temperatures are not fully established for different individuals and the effects of outdoor temperatures on those indoor temperatures may vary widely between different locations in the same building. As such, this work highlights the importance of monitoring temperatures in real-time during EHEs, especially in older healthcare facilities where potentially susceptible patients housed in different parts of the same building may be exposed to very different temperatures. Simple interventions such as highly visible digital thermometers could be potentially lifesaving. Such measurements can help staff make decisions about moving patients into cooler areas of the building if temperatures reach potentially dangerous levels. Where moving patients is not feasible, this information may also help prioritize areas for placement of portable mechanical cooling devices.
This study had several limitations. First, we did not have information on the behaviours of room occupants (e.g., opening windows, closing blinds) or on the specific reasons why different rooms may have been hotter than others, such as more detailed information about the building’s central air conditioning system. Second, the generalizability of our results is limited because we only examined temperatures in a single facility. Although this facility had central air conditioning, we still observed significant variation between floors and between rooms on the same floor. Buildings without air conditioning or newer buildings with recently installed heating, ventilation, and air conditioning (HVAC) systems may experience significantly more or less temperature variability, respectively, which should be explored in future research. Finally, these EGGs were installed as a part of another study to understand wildfire smoke infiltration. As a result, we have limited information on why specific locations were chosen and on differences between locations. Future research should deploy more sensors and collect more detailed information on differences between rooms and occupant behaviors to more fully understand the effect of extreme heat within a given facility.
Conclusions
The 2021 EHE in BC affected temperatures across an older inpatient healthcare building, with impacts varying between floors and between rooms on the same floor. This study demonstrates the utility of actively monitoring temperatures in multiple locations across older healthcare facilities to understand how people located in different parts of the building are exposed to heat in real-time. This information can be used by healthcare providers to make real-time decisions to protect the health of their patients and staff during EHEs.
Acknowledgments
Funding: This study was funded by Vancouver Coastal Health (Internal Project No. D049-00) and Health Canada (MOA No. 4500415138).
Footnote
Peer Review File: Available at https://jhmhp.amegroups.com/article/view/10.21037/jhmhp-23-81/prf
Conflicts of Interest: All authors have completed the ICMJE uniform disclosure form (available at https://jhmhp.amegroups.com/article/view/10.21037/jhmhp-23-81/coif). The authors have no conflicts of interest to declare.
Ethical Statement: The authors are accountable for all aspects of the work in ensuring that questions related to the accuracy or integrity of any part of the work are appropriately investigated and resolved. This study did not involve collecting data from participants, so it was not necessary to obtain informed consent.
Open Access Statement: This is an Open Access article distributed in accordance with the Creative Commons Attribution-NonCommercial-NoDerivs 4.0 International License (CC BY-NC-ND 4.0), which permits the non-commercial replication and distribution of the article with the strict proviso that no changes or edits are made and the original work is properly cited (including links to both the formal publication through the relevant DOI and the license). See: https://creativecommons.org/licenses/by-nc-nd/4.0/.
References
- Kovats RS, Hajat S. Heat stress and public health: a critical review. Annu Rev Public Health 2008;29:41-55. [Crossref] [PubMed]
- Lippmann SJ, Fuhrmann CM, Waller AE, et al. Ambient temperature and emergency department visits for heat-related illness in North Carolina, 2007-2008. Environ Res 2013;124:35-42. [Crossref] [PubMed]
- Ebi KL, Capon A, Berry P, et al. Hot weather and heat extremes: health risks. Lancet 2021;398:698-708. [Crossref] [PubMed]
- Walikewitz N, Jänicke B, Langner M, et al. Assessment of indoor heat stress variability in summer and during heat warnings: a case study using the UTCI in Berlin, Germany. Int J Biometeorol 2018;62:29-42. [Crossref] [PubMed]
- Mangkuto RA, Rohmah M, Asri AD. Design optimisation for window size, orientation, and wall reflectance with regard to various daylight metrics and lighting energy demand: A case study of buildings in the tropics. Applied Energy 2016;164:211-9. [Crossref]
- Lundgren Kownacki K, Gao C, Kuklane K, et al. Heat Stress in Indoor Environments of Scandinavian Urban Areas: A Literature Review. Int J Environ Res Public Health 2019;16:560. [Crossref] [PubMed]
- Vandentorren S, Bretin P, Zeghnoun A, et al. August 2003 heat wave in France: risk factors for death of elderly people living at home. Eur J Public Health 2006;16:583-91. [Crossref] [PubMed]
- Loughnan M, Carroll M, Tapper NJ. The relationship between housing and heat wave resilience in older people. Int J Biometeorol 2015;59:1291-8. [Crossref] [PubMed]
- Winters W, Summers D. Climate Projections For Metro Vancouver. Metro Vancouver. Available online: https://retooling.ca/wp-content/uploads/2020/10/Climate_Projections_For_Metro_Vancouver.pdf
- Philip SY, Kew SF, van Oldenborgh GJ, et al. Rapid attribution analysis of the extraordinary heat wave on the Pacific coast of the US and Canada in June 2021. Earth Syst Dyn 2022;13:1689-713. [Crossref]
- White RH, Anderson S, Booth JF, et al. The unprecedented Pacific Northwest heatwave of June 2021. Nat Commun 2023;14:727. [Crossref] [PubMed]
- Lee MJ, McLean KE, Kuo M, et al. Chronic Diseases Associated With Mortality in British Columbia, Canada During the 2021 Western North America Extreme Heat Event. Geohealth 2023;7:e2022GH000729.
- Henderson S, McLean K, Lee M, et al. Extreme heat events are public health emergencies. B C Med J 2021;63:366-7.
- Nguyen PDM, Martinussen N, Mallach G, et al. Using Low-Cost Sensors to Assess Fine Particulate Matter Infiltration (PM(2.5)) during a Wildfire Smoke Episode at a Large Inpatient Healthcare Facility. Int J Environ Res Public Health 2021;18:9811. [Crossref] [PubMed]
- Station Description: Vancouver Clark Drive Met. [accessed February 27, 2023]. Available online: https://envistaweb.env.gov.bc.ca/StationDetails.aspx?ST_ID=577
- Franck U, Krüger M, Schwarz N, et al. Heat stress in urban areas: Indoor and outdoor temperatures in different urban structure types and subjectively reported well-being during a heat wave in the city of Leipzig. Meteorologische Zeitschrift. 2013;22:167-77. [Crossref]
- Quinn A, Kinney P, Shaman J. Predictors of summertime heat index levels in New York City apartments. Indoor Air 2017;27:840-51. [Crossref] [PubMed]
- Dahlblom M, Jensen L. Vertical temperature increase in multi-storey buildings. 10th Nordic Symposium on Building Physics, 15-19 June 2014; Lund, Sweden. 2014:814-21.
- Gaffin SR, Rosenzweig C, Khanbilvardi R, et al. Variations in New York city’s urban heat island strength over time and space. Theor Appl Climatol 2008;94:1-11. [Crossref]
- Gerçek D, Güven İT, Oktay İÇ. Analysis of the intra-city variation of urban heat island and its relation to land surface/cover parameters. ISPRS Annals of the Photogrammetry, Remote Sensing and Spatial Information Sciences; Volume III-8, 2016 XXIII ISPRS Congress; 12–19 July 2016; Prague, Czech Republic. 2016:123-9.
- Foucquier A, Robert S, Suard F, et al. State of the art in building modelling and energy performances prediction: A review. Renewable and Sustainable Energy Reviews 2013;23:272-88. [Crossref]
- Wang Z, Chen Y. Data-driven modeling of building thermal dynamics: Methodology and state of the art. Energy and Buildings 2019;203:109405. [Crossref]
- Kenny GP, Flouris AD, Yagouti A, et al. Towards establishing evidence-based guidelines on maximum indoor temperatures during hot weather in temperate continental climates. Temperature (Austin) 2019;6:11-36. [Crossref] [PubMed]
- Meade RD, Akerman AP, Notley SR, et al. Body temperature and cardiovascular regulation in older adults during 8-hour exposures to ambient conditions experienced indoors during extreme heat events: A randomized crossover trial. FASEB J 2022; [Crossref]
- Glen P. Kenny. Extreme heat events and overheating in the home – what is a safe indoor temperature limit. Presented at the: June 29, 2022. [accessed November 25, 2022]. Available online: https://ncceh.ca/sites/default/files/BCCDC%20June%2029%202022%20Kenny%20final%20submitted%20version.pdf
- Ninomura P, Hermans R. ANSI/ASHRAE/ASHE Standard 170-2008: Ventilation Standard For Health Care Facilities. ASHRAE J 2008;50:52-6.
- ASHRAE. Chapter 9--Healthcare Facilities. In: 2019 ASHRAE Handbook--HVAC Applications. Available online: https://www.ashrae.org/file%20library/technical%20resources/covid-19/i-p_a19_ch09_health_care_facilities.pdf
Cite this article as: Dickson JM, Lee MJ, Jones K, Ebrahimi G, Henderson SB. Monitoring temperature variability inside a healthcare facility during an extreme heat event using low-cost sensors. J Hosp Manag Health Policy 2023;7:22.